Injections are never a pleasant ordeal. Children are famously scared of injections and needles and are a hassle to bring to the doctors for a jab. There are many among us who still squirm at the thought of injections, well into adulthood. However, recent advances in the way we transport drugs into our bodies might help alleviate this fear, thanks to novel drug delivery systems.
A drug delivery system (DDS) is defined as a formulation or a device that enables the introduction of a therapeutic substance in the body and improves its efficacy and safety by controlling the rate, time, and place of release of drugs in the body.(1) DDSs have existed for quite some time. In 1952, Smith Klein Beecham constructed the first sustained-release drug delivery system that controlled the drug release kinetics and achieved an efficacy of 12 hours.(2) This differed from previous methods of drug formulations, where the drug would be released immediately after coming into contact with water.(2)
The development of DDSs between the 1950s and the 1980s are classified as the first generation, where researchers elucidate many drug release mechanisms, such as dissolution, diffusion, and osmosis.(2) These developments lay the foundations of numerous oral delivery systems that are in use today.(2) The second generation of drug delivery systems, developed between the 1980s and the 2010s, focused more on creating a zero-order release system, where there is a constant drug release from the device.(3) DDSs that made use of polymers and hydrogels, that responded to stimuli, such as pH and temperature were also utilized.(2) A notable clinical product developed in this era would be the Zoladex depot, which delivered goserelin acetate over 1 to 3 months.(3) The past two generations of DDSs have addressed physiochemical barriers such as water solubility and cell permeability, but the third generation of DDSs must also overcome the biological barriers, such as the blood-brain barrier to truly succeed in pushing the boundaries of how drugs are delivered.(2,3)
Novel drug delivery systems (NDDS) come in many different shapes and sizes. One type of these NDDSs is a liposome-based delivery system.(4) Liposomes are "closed bilayer phospholipids systems", which is "fabricated from the self-assembly of phospholipids, that consist of a polar phosphate head and a hydrophobic lipid tail".(4) Liposomes are a prominent NDDS due to a few key reasons: they are easy to produce, can encapsulate a variety of drugs and are biocompatible.(4) While liposomes have existed for some time, being pioneered by Bangham et al. in 1965, where they first described "swollen phospholipid systems that established the basis for model membrane system", they have evolved in many aspects since their inception.(4–6) For example, "classical" liposomes had the problem of being taken up by phagocytes before reaching their target, but a paper by Abuchowski et al. showed that attaching polyethylene glycol (PEG) to the liposomes would increase their circulation half-life in the bloodstream.(4,7) Liposomes have also been altered so that they can unload their "cargo" when in contact with an external stimulus.(4,7) For example, ThermoDox is a "liposomal doxorubicin formulation that releases drugs in response to a mild hyperthermic trigger", where doxorubicin is an anti-cancer drug.(4) Other than delivering traditional therapeutic drugs, liposomes have also been utilized to deliver nucleic acid-based therapeutics such as antisense oligonucleotides and small interfering RNAs (siRNA).(4) Basha et al. have successfully shown that siRNA liposome NDDSs could silence the GAPDH gene inside of primary bone marrow macrophages and dendritic cells both in vivo and in vitro.(4,8)
Polymeric micelles are structures "composed of an external hydrophilic shell and central hydrophobic core suitable for incorporating water-insoluble drugs", and another carrier-based system.(9) In principle, the hydrophilic shell protects the central core from the immune system, which makes them particularly suitable to transport hydrophobic drugs.(9,10) Their size can also be controlled to be big enough to evade premature filtration while small enough to travel to its target.(10) Polymeric micelles can use different types of polymers so that it can be tailor-made to suit the situation it is in.(10) For example, polymers such as poly(lactic-co-glycolic acid) and poly(ε-caprolactone) are used to construct a hydrophobic core, and they have the added benefit of being approved by the FDA for human biomedical applications.(10) The hydrophilic outer layer can be composed of polymers such as PEG, which has "low bio-fouling and bio-inertness", as well as increasing their circulation time in the bloodstream.(10) Polymeric micelles tended to have issues with their stability, but research has been undergoing to fix this exact problem.(10,11) For example, Watanabe et al. showed that when constructing various PEG-poly(aspartate ester) block copolymer, a polymeric micelle with 5000MW of PEG and 27 aspartate ester units had the most stability.(10,12) This can be explained by the fact that the additional ester bonds can protect the original ester bonds from esterase enzymes, enzymes inside the bloodstream that break down ester bonds, which would increase their circulation time in the bloodstream.(10) A handful of these polymeric micelles have reached clinical trials, although there seem to be fewer successful products compared to liposomes.(10) For example, BIND-014 is a "docetaxel loaded PEG-PLA (poly(lactic acid))-based polymeric micelle encapsulating docetaxel, formulated as an injectable colloid which completed a clinical trial for prostate cancer (Phase-II)", and the manufacturers boast an 80-fold increase in cellular binding due to its active targeting properties.(10,13) NK-105 is a “novel paclitaxel-loaded micellar formulation encapsulated in PEG-P(Asp) with 4-phenyl -1 butanol” and was developed to reduce the risk of hypersensitivity and anaphylactic shock compared to previous drugs.(10,14)
Other than liposomes and polymeric micelles, inorganic nanoparticles also present a promising solution.(15) Inorganic nanoparticles, as the name suggests, refer to nanoparticles composed of materials such as gold, iron, and silica.(15) These particles are "precisely formulated and can be engineered to have a wide variety of sizes, structures, and geometries " and "have unique physical, electrical, magnetic and optical properties, due to the properties of the base material itself".(15) Gold nanoparticles are particularly well studied in specific areas such as photothermal therapy.(15) Photothermal therapy is a "promising biomedical application in cancer treatment", that "employs photothermal agents to with high photothermal conversion efficacy for converting light into heat to selectively kill cancer cells under the help of lasers".(16) Gold nanoparticles are a promising due to a few characteristics: high biocompatibility, easy to modify the surface of the nanoparticle, "the ability to conjugate with other drugs through gold-thiol bioconjugation chemistry" and no photobleaching.(15,16) A study by Yavuz et al. investigated how doxorubicin-filled gold nanocages would affect breast cancer cells in vitro.(15,17) The study found that the cell death rate increased with increasing exposure time to a laser, due to the increased release of doxorubicin from the cages.(15,17) Iron oxides are another inorganic nanoparticle that is well characterized for drug delivery.(15) The iron oxide nanoparticles that are researched are superparamagnetic at particular sizes, and "have shown success as contrast agents, drug delivery vehicles and thermal-based therapeutics".(15) Aval et al. created a mesoporous hydroxyapatite-coated iron oxide nanoparticle and loaded it with doxorubicin to see its drug delivery capability under different pHs.(18) The iron oxide nanoparticles released around 70% of their load at a pH of 5.5 (the pH of cancer cells) compared to 10% released at a pH of 7.4(the pH of human blood).(18)
NDDSs show a glimpse of what the future holds for cutting-edge healthcare. Many of these systems have massive potential to further improve how drugs are delivered in the body, from improving the efficacy of the drug itself to lowering the difficulty of administering the drug. Liposomes, polymeric micelles, and inorganic nanoparticles all show promising results in their respective areas, meaning different NDDSs can be utilized for areas that suit their niche. While more clinical trials are necessary to solidify their place, the research paints an optimistic future for NDDSs.
References
1. Jain KK. An Overview of Drug Delivery Systems. Methods Mol Biol [Internet]. 2020 [cited 2021 Aug 18];2059:1–54. Available from: https://link.springer.com/protocol/10.1007/978-1-4939-9798-5_1
2. Park K. Controlled drug delivery systems: Past forward and future back. J Control Release. 2014 Sep 28;190:3–8.
3. Yun YH, Lee BK, Park K. Controlled Drug Delivery: Historical perspective for the next generation. J Control Release. 2015 Dec 10;219:2–7.
4. Allen TM, Cullis PR. Liposomal drug delivery systems: From concept to clinical applications. Adv Drug Deliv Rev. 2013 Jan 1;65(1):36–48.
5. Bangham AD, Horne RW. Negative staining of phospholipids and their structural modification by surface-active agents as observed in the electron microscope. J Mol Biol. 1964 Jan 1;8(5):660-IN10.
6. Almeida B, Nag OK, Rogers KE, Delehanty JB. Recent Progress in Bioconjugation Strategies for Liposome-Mediated Drug Delivery. Molecules [Internet]. 2020 Dec 1 [cited 2021 Aug 19];25(23). Available from: /pmc/articles/PMC7730700/
7. Abuchowski A, McCoy JR, Palczuk NC, van Es T, Davis FF. Effect of covalent attachment of polyethylene glycol on immunogenicity and circulating life of bovine liver catalase. J Biol Chem. 1977 Jun 10;252(11):3582–6.
8. Basha G, Novobrantseva TI, Rosin N, Tam YYC, Hafez IM, Wong MK, et al. Influence of Cationic Lipid Composition on Gene Silencing Properties of Lipid Nanoparticle Formulations of siRNA in Antigen-Presenting Cells. Mol Ther [Internet]. 2011 [cited 2021 Aug 19];19(12):2186. Available from: /pmc/articles/PMC3242662/
9. Faheem AM, Abdelkader DH. Novel drug delivery systems. Eng Drug Deliv Syst. 2020 Jan 1;1–16.
10. Majumder N, G Das N, Das SK. Polymeric micelles for anticancer drug delivery. https://doi.org/104155/tde-2020-0008 [Internet]. 2020 Sep 15 [cited 2021 Aug 19];11(10):613–35. Available from: https://www.future-science.com/doi/abs/10.4155/tde-2020-0008
11. Cagel M, Tesan FC, Bernabeu E, Salgueiro MJ, Zubillaga MB, Moretton MA, et al. Polymeric mixed micelles as nanomedicines: Achievements and perspectives. Eur J Pharm Biopharm. 2017 Apr 1;113:211–28.
12. Watanabe M, Kawano K, Yokoyama M, Opanasopit P, Okano T, Maitani Y. Preparation of camptothecin-loaded polymeric micelles and evaluation of their incorporation and circulation stability. Int J Pharm. 2006 Feb 3;308(1–2):183–9.
13. Banerjee A, Chatterjee K, Madras G. Enzymatic degradation of polymers: a brief review. http://dx.doi.org/101179/1743284713Y0000000503 [Internet]. 2014 [cited 2021 Aug 19];30(5):567–73. Available from: https://www.tandfonline.com/doi/abs/10.1179/1743284713Y.0000000503
14. Farokhzad OC, Cheng J, Teply BA, Sherifi I, Jon S, Kantoff PW, et al. Targeted nanoparticle-aptamer bioconjugates for cancer chemotherapy in vivo. Proc Natl Acad Sci [Internet]. 2006 Apr 18 [cited 2021 Aug 15];103(16):6315–20. Available from: https://www.pnas.org/content/103/16/6315
15. Mitchell MJ, Billingsley MM, Haley RM, Wechsler ME, Peppas NA, Langer R. Engineering precision nanoparticles for drug delivery. Nat Rev Drug Discov [Internet]. 2021 Feb 1 [cited 2021 Aug 19];20(2):1. Available from: /pmc/articles/PMC7717100/
16. Yang W, Liang H, Ma S, Wang D, Huang J. Gold nanoparticle based photothermal therapy: Development and application for effective cancer treatment. Sustain Mater Technol. 2019 Dec 1;22:e00109.
17. Yavuz MS, Cheng Y, Chen J, Cobley CM, Zhang Q, Rycenga M, et al. Gold nanocages covered by smart polymers for controlled release with near-infrared light. Nat Mater 2009 812 [Internet]. 2009 Nov 1 [cited 2021 Aug 17];8(12):935–9. Available from: https://www.nature.com/articles/nmat2564
18. Abbasi Aval N, Pirayesh Islamian J, Hatamian M, Arabfirouzjaei M, Javadpour J, Rashidi MR. Doxorubicin loaded large-pore mesoporous hydroxyapatite coated superparamagnetic Fe3O4 nanoparticles for cancer treatment. Int J Pharm. 2016 Jul 25;509(1–2):159–67.
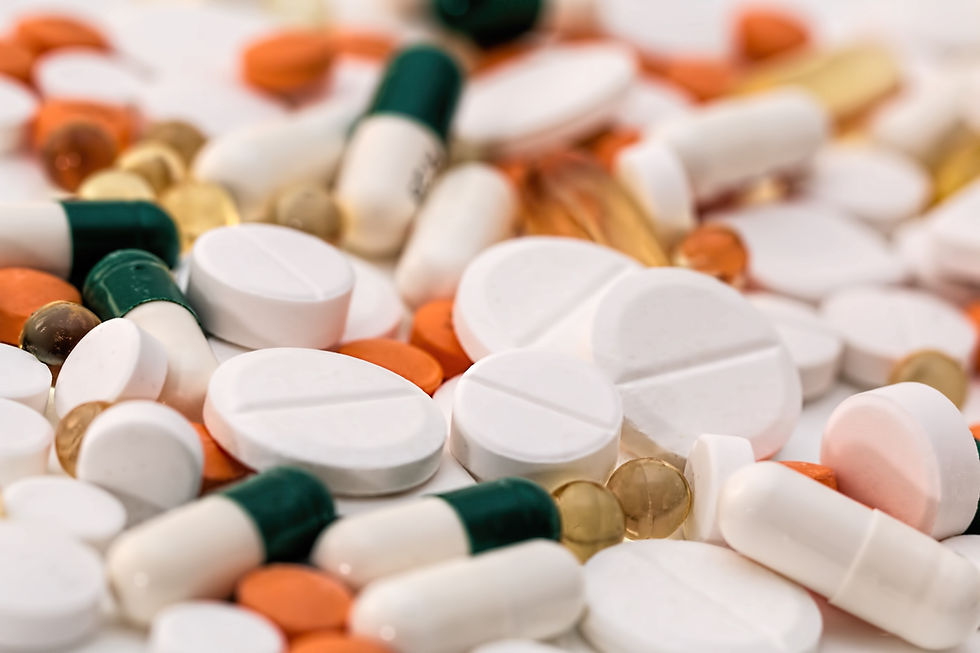
Commentaires