Silk has long been a valuable commodity, being a luxury material in ancient times and has contributed to the construction of a series of trade routes covering half the Eurasian continent. (1) While the silk acquired from silkworms (Bombyx mori) is well known, there is a lesser-known but equally interesting material: spider silk.(1) Silks are defined as “secreted proteins spun into fibrous structures” that are “produced by several arthropods such as silkworms and spiders”.(2,3) Silkworm silk is a very useful material as it possesses many special properties such as biocompatibility and low biodegradability, making it a suitable for many uses such as cloths, surgical sutures and composite materials.(4) While being lesser-known, spider silk has the added property of possessing strength and toughness, being up to 3 times as tough as Kevlar. (5,6) While silkworm silk has been studied extensively and has been mass-produced for a long time, the same cannot be said about spider silk. This is mainly due to the fact that spiders are highly territorial and cannibalistic, making them hard to keep in large numbers, unlike silkworms.(7) To overcome this problem, researchers have been vying to create an artificial method to mass-produce spider silk for several decades.
In most of the methods used in creating artificial spider silk, the DNA sequence of the spider silk is inserted into a host cell so that it can be expressed.(8) One of the most used host cells is E. Coli. A study by Xia et al. utilized a high cell density cultivation of recombinant E. coli cells to artificially create spider silk proteins.(9) The study specifically looked at 96-mer proteins (proteins with 96 repeats of the monomer) and found that its strength was 508±108 MPa, comparable to values from native dragline silk, while the stiffness was 21±4 GPa, twice the value of native dragline silk. A study by Andersson et al. also used E.coli as a host cell to express recombinant spider silk proteins.(7) This study used the fact that pH in the spider silk gland gradually decreases, and that the spider silk proteins are dehydrated through a narrowing spider silk gland and used that information to spin the silk in a technique that would mirror its natural counterpart.(7) The results showed that the artificial spider silk had a strength of 162±8 MPa and a toughness of 45±7 MJ/m3.(7) While the strength is not that hard compared to other materials, the toughness is on par with that of Kevlar and over 8 times that of high tensile steel. (5) Another host cell that has been used to create artificial spider silk proteins is P. pastoris or baker’s yeast. Fahnestock et al. conducted research in producing spider silk proteins in both E. coli and P. pastoris, where they found that homogeneity and yield of higher molecular weight silk proteins were lower in E. coli samples compared to P. pastoris samples as the E. coli cells would perform truncated synthesis(ending the synthesis prematurely).(10)Adding this to the fact that P. pastoris can excrete their product, using yeast as the host cell could simplify the process of designing the recombinant gene segment as well as the purification process when mass-production begins.(10) Another possible host cell for artificial spider silk production is mammalian cells. A paper by Lazaris et al. used bovine mammary epithelial alveolar cells and baby hamster kidney cells to express spider cell genes, where the resulting artificial spider silk had a tenacity value of 1.65 grams per denier and a maximum toughness od 173 grams per denier.(11) While the tenacity was lower than that of natural spider silk, the toughness was within range of natural spider silk toughness values. (11) Since this paper only spun one spider silk protein out of multiple other proteins, combining the other proteins might increase the artificial spider silk’s material properties.
Other than differing host cells, one can also vary the spinning technique for more favorable results. While the studies above which produced artificial spider silk by a technique know as “wet spinning”, where “the silk protein solution is extruded through a spinneret directly into a coagulation bath that initiates solidification into fiber via precipitation”, there is also another technique called ”dry spinning” where “the solidification of the fiber occurs due to the evaporation of a volatile solvent”.(12) In a study by Luo et al., a regenerated silk fibroin (silk proteins extracted from natural silk fibers) solution was prepared and injected through a microchip, and then immersed in an aqueous ethanol solution which was then passed through the microchip and immersed into the ethanol solution again. (12,13) The results showed that the strength and stiffness were 614 MPa and 19 GPa respectively, which were comparable to values of natural spider silk.(13) Another study by Ling et al. prepared a similar regenerated silk fiber solution and spun from a syringe.(14) The results showed that the stiffness was 8 ± 1 GPa and that the toughness was 14 ± 9 MJ m−3, where the stiffness was within the bounds of typical natural spider silk but the toughness was lacking.(12,14)
Other than the above-mentioned techniques, many other innovative techniques are being studied to create artificial spider silk such as electrospinning or using multicellular host cells.(15,16) However, even with the use of such innovative techniques, the mass-production of artificial spider silk has not yet come to fruition. One potential way this could be achieved, as stated in the paper by Poddar et al., would be to use directed evolution techniques.(8) Directed evolution, as the name suggests, is a technique that “optimizes natural template proteins for specific applications”.(8,17) An example of this would be the use of polymerase chain reactions in creating multiple copies of a gene with slight mutations which could result in a beneficial trait in the spider silk protein once it is expressed. Another example would be the use of DNA shuffling, a technique where “experiments generally start with many similar DNA sequence that encodes for the same functional protein, and then the sequences are then digested with DNAse 1 endonuclease enzymes to create many short fragments, which are then reassembled randomly into chimeras containing sequences from several original”. (17) This technique can also trigger relevant mutations in the gene resulting in easier mass-production.
Other than the lack of mass-production, another concern is the imprecise results of the mechanical properties of the silk. In many of the studies mentioned above, the mechanical testing of the silks did not seem to present grouped results.(9,10) This is quite detrimental when producing on an industrial scale, as a lack of reproducibility could result in a large chunk of the product being discarded due to them not reaching the required standard. A possible solution to produce spider silks with more consistent mechanical properties is to combine existing techniques. For example, the paper by Xia et al. had very favorable results in terms of its strength and stiffness.(9) Combining this method with this method of spider silk protein production and a biomimetic method of other papers, such as the one employed in the study by Andersson et al., could potentially increase the mechanical properties of the final product.(7) However complicating the production process could further hinder the mass-production of spider silk.
While spider silk remains a very alluring material with spectacular properties, there are multiple obstacles to overcome before it can be readily available for consumers to use around the world. However, the amount of research in artificial spider silk production has been growing for the last decade and there is hope for a viable method of mass-producing artificial spider silk in the near future.
References
1. Liu X. The Silk Road in World History. Oxford University Press; 2010.
2. Kaplan DL, Scheibel T. Recombinant Silk Production in Bacteria. In: Reference Module in Materials Science and Materials Engineering. Elsevier; 2017.
3. Breslauer DN, Kaplan DL. Silks. In: Reference Module in Materials Science and Materials Engineering. Elsevier; 2016.
4. Chen S, Liu M, Huang H, Cheng L, Zhao HP. Mechanical properties of Bombyx mori silkworm silk fibre and its corresponding silk fibroin filament: A comparative study. Mater Des. 2019 Nov 5;181:108077.
5. Agnarsson I, Kuntner M, Blackledge TA. Bioprospecting finds the toughest biological material: Extraordinary silk from a giant riverine orb spider. PLoS One [Internet]. 2010 [cited 2020 Jun 18];5(9):1–8. Available from: /pmc/articles/PMC2939878/?report=abstract
6. Gosline JM. The mechanical design of spider silks. 1999.
7. Andersson M, Jia Q, Abella A, Lee XY, Landreh M, Purhonen P, et al. Biomimetic spinning of artificial spider silk from a chimeric minispidroin. Nat Chem Biol. 2017 Mar 1;13(3):262–4.
8. Poddar H, Breitling R, Takano E. Towards engineering and production of artificial spider silk using tools of synthetic biology. Eng Biol. 2020 Mar 1;4(1):1–6.
9. Xia XX, Qian ZG, Ki CS, Park YH, Kaplan DL, Lee SY. Native-sized recombinant spider silk protein produced in metabolically engineered Escherichia coli results in a strong fiber. Proc Natl Acad Sci U S A [Internet]. 2010 Aug 10 [cited 2020 Jun 20];107(32):14059–63. Available from: https://www.pnas.org/content/107/32/14059
10. Fahnestock SR, Yao Z, Bedzyk LA. Microbial production of spider silk proteins. Vol. 74, Reviews in Molecular Biotechnology. Elsevier; 2000. p. 105–19.
11. Lazaris A, Arcidiacono S, Huang Y, Zhou JF, Duguay F, Chretien N, et al. Spider silk fibers spun from soluble recombinant silk produced in mammalian cells. Science (80- ) [Internet]. 2002 Jan 18 [cited 2020 Jun 22];295(5554):472–6. Available from: https://science.sciencemag.org/content/295/5554/472
12. Guo C, Li C, Mu X, Kaplan DL. Engineering silk materials: From natural spinning to artificial processing [Internet]. Vol. 7, Applied Physics Reviews. American Institute of Physics Inc.; 2020 [cited 2020 Jun 22]. p. 011313. Available from: https://aip.scitation.org/doi/abs/10.1063/1.5091442
13. Luo J, Zhang L, Peng Q, Sun M, Zhang Y, Shao H, et al. Tough silk fibers prepared in air using a biomimetic microfluidic chip. Int J Biol Macromol. 2014 May 1;66:319–24.
14. Ling S, Qin Z, Li C, Huang W, Kaplan DL, Buehler MJ. Polymorphic regenerated silk fibers assembled through bioinspired spinning. Nat Commun [Internet]. 2017 Dec 1 [cited 2020 Jun 22];8(1):1–12. Available from: https://www.nature.com/articles/s41467-017-00613-5
15. Li C, Vepari C, Jin HJ, Kim HJ, Kaplan DL. Electrospun silk-BMP-2 scaffolds for bone tissue engineering. Biomaterials. 2006 Jun 1;27(16):3115–24.
16. Teulé F, Cooper AR, Furin WA, Bittencourt D, Rech EL, Brooks A, et al. A protocol for the production of recombinant spider silk-like proteins for artificial fiber spinning. Nat Protoc [Internet]. 2009 [cited 2020 Jun 22];4(3):341–55. Available from: https://pubmed.ncbi.nlm.nih.gov/19229199/
17. Kan A, Joshi NS. Towards the directed evolution of protein materials. MRS Commun [Internet]. 2019 Jun 1 [cited 2020 Jun 23];9(2):441–55. Available from: /core/journals/mrs-communications/article/towards-the-directed-evolution-of-protein-materials/49942557F42AAAAF804A7F31330391A8
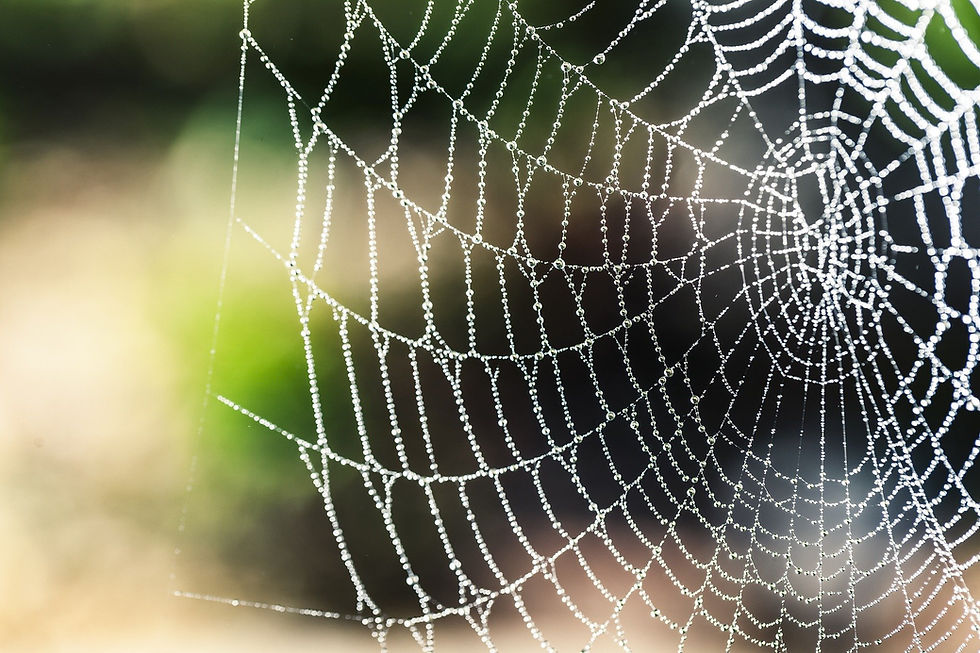
Comments